Kidney Dialysis Simulation Lab Activity Answers To Math
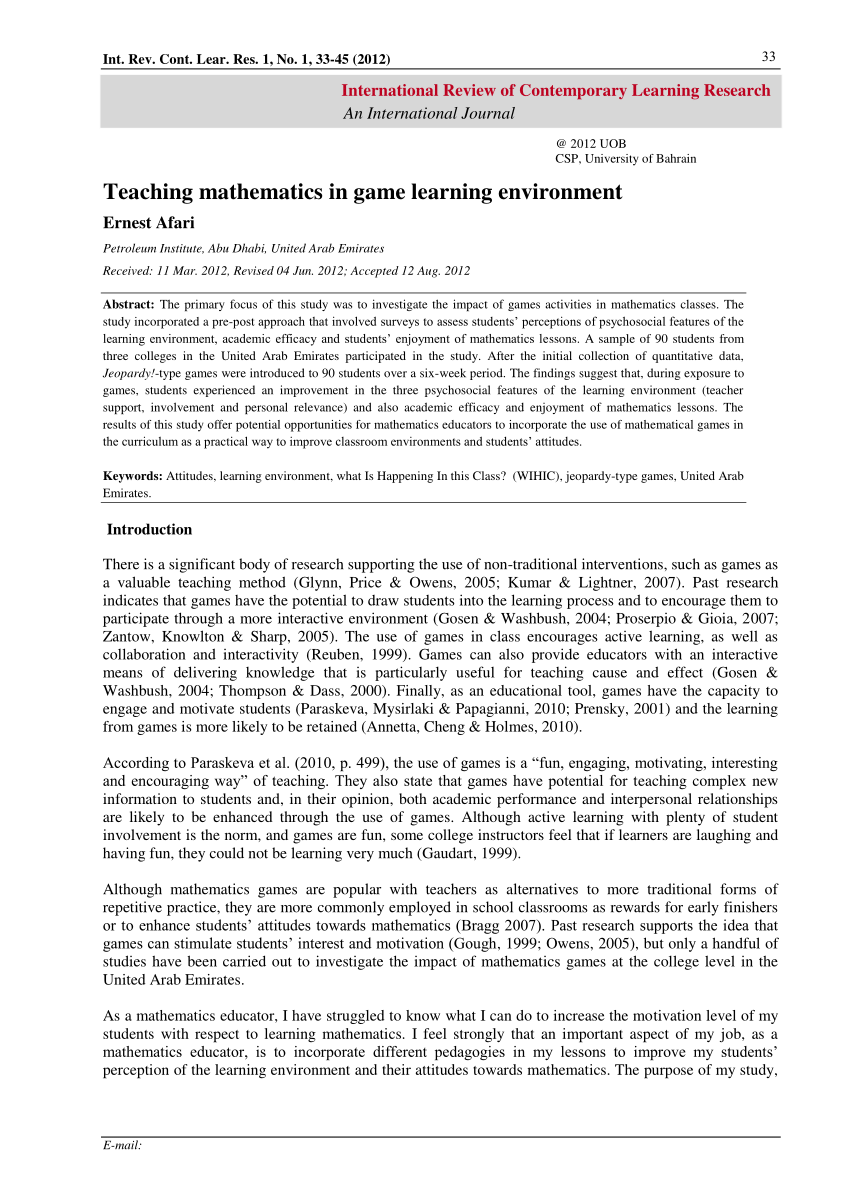
The National Kidney Foundation’s Kidney Disease Outcomes Quality Initiative (KDOQI) has provided evidence-based guidelines for all stages of chronic kidney disease (CKD) and related complications since 1997. The 2015 update of the KDOQI Clinical Practice Guideline for Hemodialysis Adequacy is intended to assist.
- Login
- About the Initiative
- Curricular Resources
- Publications
- League of Institutes
- Contact
Have a suggestion to improve this page?
To leave a general comment about our Web site, please click here
Share this page with your network.
The idea of an artificial kidney, or any artificial organ for that matter, seems like such a complex idea only to be understood by those that have specialized in fields such as nephrology or biomedical engineering. The kidney is such a complex organ. How can its function be duplicated, let alone explained to medical laypeople? Amazingly, kidney dialysis, which for all practical purposes is an artificial kidney that functions in removing solutes and toxins from the blood, is actually not as complex as one might imagine. In fact, the mechanism by which dialysis works involves simple diffusion and osmosis, which is generally taught to children in the United States as early as middle school. The study of artificial organs can seem intimidating and beyond the comprehension of those with only a general knowledge of science. However, the study of artificial kidneys provides an amazing opportunity to display how even the most fundamental and seemingly simple concepts in science can be used to revolutionize modern medicine. Imagine a middle schooler's, or even a high schooler's excitement, when they realize they have the capability and background knowledge to create their own version of kidney dialysis.
The purpose of this unit is to teach students about the cellular processes of osmosis and diffusion. The concept of osmosis and diffusion is vital to understanding the nature of organisms and how they function, yet these have also proven to be among the most difficult concepts to get students to understand. In my experience, osmosis and diffusion are taught in isolation, generally using one cell and the surrounding environment as the ultimate reference point. It is hard for a student to understand the importance of equilibrium, when the process of diffusion is not linked to an idea bigger than a small two-dimensional drawing of a circle on paper to represent a cell. This is usually shown with an arrow pointing in the direction that water will flow in relation to the cell membrane.
The purpose behind this unit is to show how osmosis and diffusion are vital to the health of entire systems and organisms; it is not a rare occurrence that just happens in cells that have been isolated. In teaching kidney function, students will get to connect what is happening on the cellular level to what is happening within the entire organ system and then the entire human body. This will allow students to truly understand homeostasis and equilibrium as it relates to their own health and the state of their own bodies. In doing so, students will be able to see the importance of healthy living choices, especially as disease such as diabetes are on the rise.
The proposed unit will be designed for a middle school classroom, but can easily be adapted for high school. I am an 8 th grade science teacher in a high poverty, urban area. My students tend to test below grade level in reading, which can sometimes interfere with their success in science, so there will be a large emphasis on hands-on, inquiry-based learning to help engage students.
The Cell Membrane
The cell is the basic functional unit of all living things. If one is to understand what is happening within an organism, it is necessary to study what is happening to the individual cells within the organism 1. This unit will cover the movement of materials—mainly water, salts and sugar—into and out of the cells of the kidney through passive transport. This will call for a thorough understanding of the cell membrane that surrounds all animal and plant cells.
The cell membrane, sometimes referred to as the plasma membrane, is a phospholipid bilayer that creates a definite boundary between the inside of the cell and the outside of the cell. The structure of cell membrane allows it to easily regulate the materials that enter and exit the cell 2. The individual phospholipids that are arranged in the membrane are comprised of a head group, which is hydrophilic, and two fatty acid chains, which are hydrophobic 3. This unique structure results in the bilayer that surrounds the cell, with the fatty acid chains or tails pointing inward (tail-to-tail) and the hydrophilic heads oriented outwards, so that the portion of the membrane that surrounds the inside of the cell (the cytoplasm) and the outside of the cell (the interstitial space) are hydrophilic. The inner portion of the cell membrane, where the fatty acid chains meet up from the inside and outside layers of the cell membrane, is a hydrophobic region. This arrangement is comparable to an oreo cookie, where in order for a material to pass through the whole cookie, it would have to pass through the chocolate cookie on one side (hydrophilic head), the cream-filled center (hydrophobic fatty acid tails), and once again the chocolate cookie on the other side. Materials that are unable to pass through the cream-filled center are unable to pass through the whole cookie.
The fact that the cell membrane is lined with both hydrophobic and hydrophilic layers makes it very difficult for most materials to pass in and out of the cell. This could be compared to a factory that continually makes products, yet is unable to ship any raw goods into the factory or ship out any of the final products. Obviously, no such factory could exist. Many materials that are necessary to cell functioning, such as salts, glucose, and amino acids, are not able to permeate the cell membrane because of the phospholipid bilayer. If the cell membrane were simply made of the phospholipid bilayer described above and nothing else, it would be a logical conclusion that anything made inside of the cell would not be able to leave the cell and anything that was found outside of the cell would not be able to enter, other than a few, very small, uncharged molecules. This is not the case, however, as it common knowledge that cells produce a variety of materials, such as hormones, and send them throughout the body; likewise, molecules, such as glucose and amino acids, enter the cell to be used for energy and to build proteins, respectively. How is this possible?
Transport of essential molecules is possible because embedded in the cell membrane are proteins (or clusters of proteins) that span the thickness of the membrane and act as transport channels for materials to move selectively in and out of the cell. These proteins come in contact with both the extracellular space and the intracellular space and, like the cell membrane itself, these proteins have areas that are hydrophobic and areas that are hydrophilic. The hydrophobic region of the protein is in the center of the cell membrane (i.e. the cream of the oreo cookie mentioned above), floating amongst the hydrophobic fatty acid chains, and the hydrophilic regions hang out slightly over the edges of the cell membrane surrounded by the hydrophilic region of the cell membrane. These proteins are capable of chemically recognizing certain materials and transporting them into or out of the cell 4.
Because the cell membrane allows certain materials to pass through it, and keeps other materials out of it, it is referred to as a semi-permeable or selectively permeable membrane. Much like the security guard at a government center, movement into and out of the cell is highly regulated.
Diffusion and Osmosis
Water, carbon dioxide, and oxygen are a few substances that are able to pass through the cell membrane without any help from the proteins embedded in the membrane. These materials can simply pass through the membrane without the use of energy and they will do so in an attempt to reach an equal solute concentration inside and outside of the cell. This process is called diffusion, when substances move from a region of high concentration to a region of low concentration. The 'goal' of diffusion is to reach a state of equilibrium. This does not mean that movement of molecules stops once equilibrium is reached. Equilibrium is a state of balance, where for every molecule of a substance that moves into a cell, another one moves out of the cell 5. To visualize diffusion, imagine two rooms that are connected by one door. If the door connecting the two rooms is closed and everyone is crammed into only one of the rooms, as soon as the door opens, people will begin to walk into the other room to spread out. Most likely, they will not all move into the newly opened room. Instead, they will spread out until every area of the two rooms has roughly the same density (or concentration) of people. People will still be able to walk around and mingle, but they will likely readjust so that every part of the room is equally comfortable at all times. Diffusion is an easy concept to model. Simply place a drop of food coloring into a glass of water (being careful to make sure that the fluid in the glass is completely still). The food coloring will slowly spread out in the water until it is evenly mixed throughout 6.
Diffusion In the Human Body
Within the body, diffusion is vital to the functioning of the cardiovascular system. When oxygen is inhaled into the lungs, it ends up at high concentration in the alveoli, the round sacs at the end of the bronchioles. Because blood that is flowing through your lungs is deoxygenated, there is a higher concentration of oxygen inside the alveoli than in the blood. In an attempt to reach equilibrium, the oxygen simply diffuses into the blood across the cells at the alveolar/capillary boundary. Similarly, the blood circulating through the capillaries in your lungs contains high amounts of carbon dioxide that has been picked up as a waste product from cellular respiration. The concentration of carbon dioxide in the blood is much higher than it is in the alveoli, and the carbon dioxide moves into the alveolar gas through simple diffusion; it is then exhaled out of the body 7. In regards to cells, simple diffusion is only possible if the material is able to permeate the membrane.
The Diffusion of Water- Osmosis
Like carbon dioxide and oxygen, water is able to move across the cell membrane from areas of high concentration to low concentration. This movement is aided by the presence of small channels created by proteins, which are called aquaporins. When water diffuses across a membrane, it is referred to as osmosis 8. Often, water will move across a membrane in order to balance the unequal concentrations of a solute, which is not able to move through the membrane itself. A solute is a material that is dissolved in a liquid. For example, in salt water, the solute is the salt.
Osmosis is most easily understood by imagining an experiment. Imagine a beaker that has been divided in half by a membrane that is permeable to water and impermeable to sugar, like most cell membranes in animals. Imagine that red sugar (for the purpose of this example, you will have to pretend that the sugar itself is actually red, so that as you add it to water, it turns light pink and the more you add the darker red the water gets) has been added to side A and very little sugar has been added to side B. Nature wants things at equilibrium, and there is not a state of equilibrium between the two sides of the beaker. At this point, side A should be dark red and side B would be a very light shade of pink. The simplest solution to the non-equilibrium problem would be for the sugar to move across the membrane until half of the sugar molecules are on side B and half are on side A. However, the membrane separating the two sides does not allow sugar to pass. An alternate solution to the non-equilibrium problem is possible. Water molecules can move from one side to the other to even out the concentration of sugar and water on both sides. If one side of the beaker (side A) is red and the other side (side B) is light pink, as it has very little sugar, then equilibrium could be achieved when both sides A and B are an equal shade of pink. This can happen when water passes through the membrane from side B into side A until the solute concentration of both sides was the same. (Notice that this would, of course, decrease the volume of side B and increase the volume of side A.) Once again, this does not mean that the water would simply stop moving once both sides turned pink. It means that water would be moving at an equal rate between sides A and B. (If this unit were designed for a high school class or even a high level middle school, it would be appropriate to discuss concentration and osmotic pressure in detail, but for the unit being proposed, the descriptors 'higher concentration' and 'lower concentration' will suffice. )
Osmosis—and the need to regulate solute concentrations in organisms, or maintain homeostasis—can be demonstrated by placing cells in solutions of varying solute concentrations. Cells already have a certain level of dissolved solutes within them. If a cell is surrounded by a solution with the same level of solute concentration as the cell itself, there will be no net movement of water into or out of the cell. This solution would be referred to as an isotonic solution, meaning the concentration of solutes inside the cell and outside of the cell were the same 9.
If the same cell were surrounded by a solution that had more dissolved solutes than the cell itself, then water would leave the cell in order to reach equilibrium. Think back to the hypothetical red sugar example above. The end goal is pink, and water will flow from where the color is a darker red to where it is lighter or where there is no color at all. The darker red would be outside of the cell and the lighter pink would be inside of the cell. This would cause the volume of the cell to decrease as water left the cell. In this case, the solution surrounding the cell would be described as a hypertonic solution.
If the same cell were instead placed in distilled water or water with very few solute molecules, then water would enter the cell in an effort to reach equilibrium. This would cause the cell to expand in volume. If the concentration difference was large, so that a large volume of water had to move into the cell to equalize concentrations, this process could actually result in the cell bursting. In this case, the external solution would be described as a hypotonic solution. Because most cells have a relatively large number of solutes in their cytoplasm, maintaining an environment that is isotonic to the cell is imperative. For the paramecium, a single-celled protist usually found in freshwater, there is a constant struggle to remove water that flows into the cell in a futile effort to reach equilibrium. In order to prevent the paramecium from rupturing, the paramecium has a contractile vacuole which continually pumps the water out of organism 10.
The Kidneys
Adult kidneys, of which there are two, are approximately 3 centimeters thick, 6 centimeters wide, and 12 centimeters long 11. The kidneys lie in the back abdominal wall. Their major function is to maintain homeostasis within body and they accomplish this by filtering the blood 12. The end process of kidney function is urine, which is produced to maintain the internal body environment through the regulation of certain solutes, such as potassium and sodium ions 13.
The kidneys are arguably one of the most complex of all the organs of the human body. There is an overwhelming amount of information regarding the many different mechanisms of kidney function, including how it regulates solute concentration in urine, how it adjusts itself according to changes in the internal environment, and the physiology that explains how it all works. However, not all of this information is necessary for the proposed curriculum unit. In order to help focus on only what is necessary to teach osmosis and diffusion, only urine formation and the transport of salt, water and glucose will be discussed in detail.
The Nephron and Urine Production
The basic functional unit of the kidney is the nephron. The nephron is a tubular structure that is lined with one layer of epithelial cells. On average, each kidney is comprised of approximately one million nephrons. Blood first enters the kidneys by way of the renal artery. A large volume (1300 mL) of blood enters the kidney every minute. Upon entering the renal artery, blood then travels through the afferent arteriole, which leads into an individual nephron 14.
The Glomerulus
The afferent arteriole directs the blood into a capillary structure called the glomerulus. The glomerulus functions as a high pressure filter. As blood passes through the glomerulus, proteins and blood cells are separated from the plasma. The filtrate, a protein-free plasma solution, ends up in the Bowman's capsule of the nephron. The Bowman's capsule is a membrane bound sac that surrounds the glomerulus. The blood cells and proteins that were not filtered into the Bowman's capsule flow out of the nephron through the efferent arteriole, which carries blood out of the nephron. At this point, the fluid that has been collected in the nephron is referred to as filtrate and is the precursor to what will eventually become urine 15.
The glomerulus is essentially a capillary bed, but it has unique properties that make it possible for simple filtration to occur without the use of any energy (other than the energy that is consumed in the heart to create pressure in the blood). The first is that the pressure in the glomerular capillaries is much higher than an average capillary bed and the second is that the capillaries are much more permeable than most capillary beds 16.
The Proximal Tubule
The filtrate that is produced in the glomerulus is protein-free plasma that contains glucose, amino acids, vitamins, minerals and any other solutes that are contained in the blood. If the nephron's structure allowed for only simple filtration, as occurs in the glomerulus, all of these important chemicals necessary in the body for healthy functioning would be excreted in the urine and would not be available for use. If these molecules were lost, they would have to be continually replaced in the diet. Fortunately, the nephron is able to recover most of these useful, filtered molecules. As the filtrate moves through the remainder of the nephron, these materials are reabsorbed into the body, since a healthy kidney does not produce urine that contains glucose or many of the other solutes found in the filtrate at this early stage of urine production.
The next region of the nephron is the proximal tubule, which receives about 120 mL of filtrate per minute. The proximal tubule is the region of the nephron responsible for the reabsorption of certain solutes, especially glucose. To understand how glucose is moved out of the fluid in the tubule, and eventually reabsorbed into the blood stream by way of the peritubular capillary bed that surrounds the nephrons, it is necessary to follow the movement of sodium ions that are also present at high concentration in the filtrate. There is a high concentration of sodium ions inside of the proximal tubule because of the initial filtration process. But sodium concentrations are kept relatively low in the epithelial cells that line the proximal tubules. Because of the concentration gradient, sodium ions will move from inside the tubule, through the cells and into the interstitial space. Of course, this requires a protein to transport them out of the cell, since ions cannot pass through the lipid bilayer. Sodium ions are transported into the cells and then pumped from the cells to the interstitial space outside of the cells. The peritubular capillary bed that surrounds the nephron has a low salt concentration as well, since salt was originally removed in the glomerulus. Therefore, the sodium ions diffuse into the capillary bed and are reabsorbed. As salt concentrations increase in the interstitial space outside of the proximal tubule, osmotic pressure increases and water moves outside of the proximal tubule as well. Ultimately, this water is also reabsorbed by the peritubular capillaries 17.
Reabsorption of Glucose
Glucose, like most molecules, is not capable of simply diffusing across the cell membrane when there is a concentration gradient. In order for glucose to be reabsorbed by the body after being filtered out of the blood, glucose transport is actually coupled with the sodium ion transport, as described above. Transport proteins in the cell membrane are activated when both glucose and Na + are available. The transporters require both substances to be present and they operate without the use of any energy. As previously stated, Na + concentrations are kept low in the cells that line the proximal tubules. Therefore, sodium will continue to move down its gradient, so long as there is glucose available in order to activate the transport protein. In this mechanism, sodium is transported from the lumen of the proximal tubule into the cells that line the tubule. Once inside, the sodium and the glucose molecules are still at a higher concentration than they are in the surrounding interstitial space and the peritubular capillaries. There are specialized transport proteins on the side of the nephron cells closest to the peritubular capillaries that are capable of transporting glucose separately from hydrogen. Since glucose is moving down its concentration gradient, as is sodium, this movement of molecules is passive and requires no energy 18.
Figure 2. A. Movement of solutes and water from the tubular fluid to the blood is regulated by tubular epithelial cells. B. Tubular epithelial cells transport sodium ions from the luminal fluid to the interstitium. C. Tubular cells have co-transporters that allow glucose to be reabsorbed together with sodium. Reproduced with permission from ref. 2 (Saltzman, 2009).
The Loop of Henle and Reabsorption of Water
At this point in the journey to create urine from blood filtrate, the glucose absorbed in the filtrate has been reabsorbed by the body, as has some salt and some water. However, it is in the body's best interest for the kidney to recover as much water as possible from the filtrate, creating the most concentrated urine. In order to get water to leave the filtrate through diffusion, the area surrounding the nephron must have a high salt concentration. A high salt concentration in the interstitial fluid outside of the nephron will provide a driving force for osmosis, allowing water to be recovered from the filtrate.
The reabsorption of water occurs in many places in the nephron, but especially in the collecting duct, which is the final segment of tubule in the nephron. To allow for the reabsorption of water, the nephron needs a mechanism for creating high solute concentrations (i.e. high osmotic forces) in the fluid outside the collecting duct. This is accomplished by the Loop of Henle. If you could imagine a tube shaped like a U, this is how the Loop of Henle looks. In the descending side of the loop, the cells are water permeable. In the ascending portion of the loop, the cells are not permeable to water. The cells in the ascending tubule also contain pumps that use energy to transport salt into the interstitial fluid surrounding the Loop and collecting duct. These pumps that remove salt from the ascending loop are important because they pump NaCl into the interstitial fluid that surrounds the Loop of Henle. This helps keep the concentration of salt higher in the interstitial fluid outside of the descending loop 19. Because of the presence of high salt concentrations in the interstitial fluid, as the filtrate travels down the collecting duct—on the way out of the nephron—there is a strong driving force for water to diffuse through the cells that line the collecting duct. In these last stages of flow through the tubule, the filtrate can become extremely concentrated 20.
As osmosis continues and more water moves into the interstitial fluid surrounding the loop, the filtrate becomes much more concentrated. This is the instance of osmosis that the curriculum unit will focus on, and rather than get into specifics of osmotic pressure and the role of the ascending loop, the 'story' will be simplified and students will simply be informed that the body uses energy—within the Loop of Henle—to keep the area surrounding the Loop of Henle and collecting duct high in salt concentration, in order to encourage osmosis and the reabsorption of water by the body. Of course, this eliminates much of the more subtle detail involved in this process. If this were to be taught in a high school class, the role of the ascending loop would be appropriate to include and could also be used to further explain active transport, as opposed to the passive transport that happens more frequently in the kidney.
Tubular Secretions
The final stage of urine formation removes certain waste products from the blood that could be toxic if they were allowed to accumulate. Tubular secretions are how metabolic wastes, certain drugs, hydrogen and potassium ions and other materials end up in the urine 21. The collection of these materials occurs in the distal tubule. The filtrate now flows into the collecting duct of the nephron. As the filtrate travels through the collecting duct, certain materials may once again be reabsorbed, depending on the current conditions of the body. The mechanism for this response to the internal environment is key to understanding how the kidney maintains homeostasis 22. It is not important to this unit however, and will not be described in detail.
The Collecting Duct and Excretion of Urine
The collecting duct contains the filtrate, which is now referred to as urine. The process described above is occurring in every one of the one million nephrons in each kidney. Each collecting duct at the end of each nephron will funnel into a larger tubule. This happens repeatedly until all of the urine is funneled into the ureter 23. The ureter is a tubular structure that carries the urine from the renal pelvis to the bladder. Once urine volume in the bladder reaches a specific volume, approximately 200- 400 mL, a receptor in the wall lining of the bladder is stimulated, creating the feeling that the bladder needs to be emptied. From the bladder, urine leaves the body through the urethra 24.
Kidney Malfunction
It is the kidney that regulates the concentration of solutes in our blood and maintains the correct balance of sodium, water and other materials 25. The kidney is a finely tuned organ that responds to our constantly changing internal conditions, adjusting and re-adjusting itself to maintain homeostasis. The filtration system of the kidneys maintains the necessary ion levels in the blood. This allows our muscles to function, which in turn keeps our heart functional and allows our diaphragm to contract in order for us to breathe. Additionally, it removes the waste products our body creates, especially urea, which could lead to death if allowed to accumulate. In the event of kidney malfunction, it is not possible to regulate our own blood composition by simply being selective with our diet. When the kidney malfunctions or shuts down completely, the rest of the body soon follows suit.
There are multiple causes of renal failure today, some genetic or rare, and seemingly unpreventable. Examples of these are shock due to fluid loss after an accident or the use of certain necessary medications 26. Currently, however, more than two-thirds of all cases of kidney disease are diabetes and hypertension. Prolonged kidney disease can eventually lead to renal failure. When a person reaches end stage kidney failure, which is defined as the loss of at least 85% of kidney functionality, kidney dialysis is necessary 27.
Hemodialysis
Once a person has reached the point that their kidneys are, for all practical purposes, non-functioning, it is necessary to filter the blood through artificial means to keep the person alive. A solution to renal failure is dialysis, in which an artificial apparatus filters the blood, removing the waste products such as urea. The first dialysis machine was constructed by Dutch physician, Dr. Willem Kolff, in 1941. His machine was fashioned from the cooling system of an old Ford, cellophane wrapped sausage skin, parts from an old downed German airplane and a porcelain bathtub 28.
Today's kidney dialysis machines are obviously made a little differently than Dr. Kollf's, but the basic principle remains: remove a small amount of blood from the body at a time and filter out urea from the blood through simple diffusion. Today's dialysis, however, is able to remove more than just urea from the blood. Kidney dialysis is able to remove other waste products and balance essential ion concentrations 29.
There are now two types of dialysis: hemodialysis and peritoneal dialysis. In hemodialysis, blood is removed from the body and filtered through an artificial membrane 30. In peritoneal dialysis, the actual lining of the abdominal cavity is used as the filter and a solution is injected directly into the abdomen. As blood flows around the peritoneal cavity, diffusion of solutes from the blood occurs directly through the peritoneal membrane and then the fluid is removed later. For the purpose of this unit, only hemodialysis will be used as an example, to simplify the material.
The way that current hemodialysis works is to temporarily remove blood from the body, flowing the blood through a tube surrounded by a carefully selected permeable membrane which is surrounded by a fluid called the dialysate, and then return the filtered blood back into the body 31. Dialysis works simply because molecules naturally want to be in a state of equilibrium and will diffuse through a membrane (assuming the pores are large enough) in order to reach this state. If a hypertonic solution is surrounded by a hypotonic solution, the solute particles will diffuse across the membrane. The dialysate is a solution that has been specially formulated to remove specific materials from the blood before sending the blood back into the body. For example, ideally, little to no urea should be present in the blood when it is sent back into the body. As the blood passes through the tube, which would have pores large enough for urea to pass through, the dialysate would contain no urea. This would cause urea to move through the membrane into the dialysate, thereby reducing the concentration of urea in the blood. Of course, this is a slight oversimplification and there are multiple factors that will determine the rate of diffusion during dialysis. The rate of diffusion, and therefore the success of the dialysis, is dependent of the concentration gradient between the blood and the dialysate, the material used for the membrane and the size and properties of the solute that is diffusing 32.
Morbidity and Mortality of Kidney Dialysis
In the past twenty years there has been a dramatic increase in the prevalence of kidney disease. While kidney dialysis was a huge breakthrough in medicine, it is by no means a perfect solution for treating kidney failure. Morbidity and mortality rates are still high in patients receiving dialysis. Patients that receive dialysis have been shown to have a much shorter life expectancy than average 33.
While all strategies that are being proposed are appropriate for a middle school classroom, they have all been chosen specifically to meet the needs of high risk students that traditionally perform below grade level. The strategies have been chosen to optimize engagement and lend themselves well to differentiation. The main strategies that will be used will be: demonstrations, modeling, hands-on lab activities and problem based learning.
Demonstrations
In the middle school classroom, it is not always possible or wise to let students do certain activities on their own. Demonstrations allow for a quick 'gotcha' moment in the classroom without losing all the time with a full lab set-up. The questions that are asked by the teacher and the questions asked by the students determine the value of a demonstration.
Hands-On Labs
There is little question that hands-on learning is beneficial to students and their level of engagement. The hands-on activities being proposed will allow students to witness osmosis and diffusion and to experiment with ways to control it.
Writing Activity
Writing is usually a struggle for students, especially middle school students. In this unit, students will write a story book for small children using their notes and other background information. This will provide them with an opportunity to create their own differentiated product.
Demonstration Lab
Purpose: The purpose of this lab will be to introduce students to osmosis and diffusion using chicken eggs, since they are visible to the naked eye. This demonstration is 'the hook' that can be used as a reference point throughout the rest of the unit.
Procedures (for the teacher):
1. Soak 2 chicken eggs in vinegar for 24-48 hours in order to dissolve the shell.
2. Have students measure the mass of each egg after the shell has been removed. (To save time, the teacher could measure the mass and give the data to the students.) Have students record the mass of each egg. Be sure to point out that the egg is a very large cell and it is surrounded by a cell membrane.
3. Create 2 different beakers with each of the following solutions: water and corn syrup and distilled water.
4. Measure the mass of each solution.
5. Place 1 egg into each beaker and let them sit overnight.
6. After at least 24 hours, remove the eggs from the solution.
7. Find the mass of the eggs and the remaining solution.
Questions for students:
1. What happened to the size of the egg when placed in the corn syrup?
2. Excel for mac 2011 add percent style. What happened to the size of the egg when placed in the distilled water?
3. A hypertonic solution is one where there are more solutes compared to the inside of the cell. Which solution was hypertonic?
4. A hypotonic solution is one where there are less solutes compared to the inside of the cell. Which solution was hypotonic?
5. What could you do to make the cell (egg) get larger?
6. What could you do to make the cell (egg) get even smaller?
7. What do you think would happen if you placed the cell in really salty water?
Potato Lab
In between the egg lab and The Potato Lab, students should have some form of instruction that covers the specifics of what is able to enter and exit the cell and what equilibrium is. Prior to beginning this lab, remind students that potatoes are living, so they are made out of cells. Students may also need to be reminded that cells contain a number of solutes.
Procedure for students:
1. Cut out 2 small potato cubes that are roughly the same size. (In my classroom, I would cut these myself).
2. Find the mass of each potato cube.
3. In a beaker, pour in 100 ml of water. Add salt in order to make a saturated solution. This will be beaker 1.
4. In another beaker, pour 100 ml of distilled water. This will be beaker 2.
5. Add one potato cube to each beaker.
6. Let the potatoes sit for at least 24 hours.
7. After 24 hours, measure the mass of the two potatoes.
Pre and Post Lab Questions:
1. In which beaker is the solution hypertonic?
2. In which beaker is the solution hypotonic?
3. What happened to the mass of each potato?
4. Explain why the mass of each potato changed using what you know about osmosis.
Writing Assignment- The Story of Pee
Students will receive notes on kidney function and how pee is made. These notes will be used by students in order to make a short book explaining how pee is made. They will trace their lunch, including the water they drink, from the time they eat the food to the digested food particles entering the bloodstream and ultimately the kidneys. This activity can easily be differentiated based on the level of the students. Some students may need additional diagrams and flow charts to help them follow all of the steps.
Dialysis Lab/ Guest Speaker
Prior to this lab, students should briefly be exposed to some of the causes of kidney failure and a short explanation of kidney dialysis. I will also get a guest speaker from the community to come talk to my students. The guest speaker will be someone from a dialysis lab. This will help provide students with some of the background information necessary for understanding the lab.
In the following lab, students will experiment with a simulation of kidney dialysis, using artificial blood and a dialysate. For the purposes of my classroom, we will only focus only the salt, proteins, red blood cells and urea in the blood 34.
Procedure:
1. Students will make 'artificial blood' by combining the following ingredients in a large test tube:
1. 2 mL of table salt
2. 2 mL of 'protein' (baking soda)
3. Approximately 1 teaspoon of red glitter or sequins to represent red blood cells
4. 2 drops of yellow or orange food coloring to represent urea and other waste
5. Enough warm water to fill the test tube up
2. Place a stopper in the test tube and gently shake the 'blood'.
3. Obtain a piece of dialysis tubing. Wet one end and tie a knot.
4. Pour the 'blood' into the dialysis tubing and tie another knot at the top of the tubing so that it is sealed. This represents the blood that would be removed from the body during dialysis.
5. Place the dialysis tubing into a cup of warm distilled water and let it sit for 10 minutes.
6. At the end of the 10 minutes, test the dialysate to see if it contains each solute. Read each question below for directions on how to determine if the solute left the blood.
a. If any of the urea or waste products left the blood, the dialysate would be orange/yellow. Did the urea leave the blood?
b. Dip the salt indicator strip into the dialysate. Did the salt leave the blood?
c. Dip the chromatography paper into the dialysate. If it turns blue, protein is present. If it remains white, protein is not present. Did the protein leave the blood? (The chromatography paper will not change colors. You could also call it 'protein indicator paper'.)
d. Observe the dialysate. Did any red blood cells move through the membrane?
Post Lab Questions:
1. Why do you think the protein did not move through the membrane but the salt did?
2. Do you think all of the salt left the blood? How do you know?
3. How would you change the dialysate if you wanted more salt to remain in the blood?
Through the activities proposed above, students should gain a thorough understanding of osmosis and diffusion and how imperative these processes are to the health and well- being of organisms. In addition to teaching students cellular processes, this unit touches on some of the health issues that Americans are facing today, namely diabetes. What I hope that my students will take from this unit is not only an understanding of osmosis and diffusion, but also an appreciation for what their bodies do for them every day. It is my hope that this will encourage students to take better care of themselves and think about the long term consequences of unhealthy living.
North Carolina Goal 6.01 Describe the cell theory. According to the Standard Course of Study in North Carolina, students are expected to understand the basics of the cell theory. Students should know that cells are the basic units of structure and function. This unit gives students a relevant example of how cell structures are related to their function. This unit also provides a model for how cells provide structure and carry on major functions to sustain life.
North Carolina Goal 6.02- Analyze structures, functions and processes within animal cells. Students are expected to understand the role of cell organelles and the processes which occur inside of every animal cell. This unit teaches students about the role of the cell membrane as well as the removal of waste products.
Annotated Teacher Resources
Applegate, Edith. The Anatomy and Physiology Learning System. 1995. Reprint, Philadephia: Elsevier, Inc., 2006. This is a great book that reviews all the basics about the kidney.
Callaghan, C. A. The renal system at a glance . 2nd ed. Malden, Mass.: Blackwell Pub., 2006. This book provides specific details about kidney functioning. It is much more in depth than necessary for middle school, but still a great source of detailed information.
Friedman, Eli A., and Mary C. Mallappallil. Present and future therapies for end-stage renal disease . New Jersey: World Scientific, 2010. This was a great up-to-date analysis of the state of kidney disease treatment. It provided insight and analysis of how kidney failure has been treated and brings up some of the current issues in medicine.
Hill, Lisa. Cells biology . Chandni Chowk, Delhi: Global Media, 2007. This book provides great explanations of osmosis and diffusion and is very easy to understand.
Kapit, Wynn, Robert I. Macey, and Esmail Meisami. The physiology coloring book . 2nd ed. San Francisco: Addison Wesley Longman, 2000. This is an awesome resource for the classroom. While it is very detailed, the basic material can easily be adapted for the classroom.
University of Rochester. 'Kidney Dialysis.' Life Sciences Learning Center. lifesciences.envmed.rochester.edu/curriculum/SEPAClass/3.TEACHERKidneyDialysis7-23-09.pdf (accessed August 17, 2011). This is a great unit that focuses on teaching about kidney dialysis in more detail.
'National Kidney Foundation.' National Kidney Foundation. http://www.kidney.org/ (accessed August 10, 2011). This website provides lots of up-to-date data regarding kidney diseases. It would be a great resource for kids to use.
Rennke, Helmut G., Bradley M. Denker, and Burton David Rose. Renal pathophysiology: the essentials. 2nd ed. Philadelphia: Lippincott Williams & Wilkins, 2007. This book is written more for medical students, but it has some great sections about kidney dialysis.
Rizzo, Donald C. Fundamentals of anatomy & physiology . 2nd ed. Clifton Park, NY: Thomson Delmar Learning, 2006. This is a tool for learning more background knowledge on the kidney and its structure and function.
Saltzman, W. Mark. Biomedical engineering: bridging medicine and technology. Cambridge: Cambridge University Press, 2009. This is a magnificent resource for up-to-date material on cell biology, kidney function and dialysis.
'Willem Kolff - Telegraph.' Telegraph.co.uk - Telegraph online, Daily Telegraph and Sunday Telegraph - Telegraph. http://www.telegraph.co.uk/news/obituaries/4604625/Willem-Kolff.html (accessed July 10, 2011). This website provides some interesting biographical information about the first person to perform kidney dialysis
- 1 Donald Rizzo, Fundamentals of Anatomy and Physiology (Clifton Park: Thomson Delmar Learning, 2006), 42.
- 2 Mark Saltzman, Biomedical Engineering: Bridging Medicine and Technology (Cambridge: Cambride University Press, 2009), Chapter 5, pg 3.
- 3 Wynn Kapit, Robert I. Macey and Esmail Meisami, The Physiology Coloring Book (San Francisco: Addison Wesley Longman, 2000), 1.
- 4 Lisa Hill, Cells Biology (New Delhi: Global Media, 2007), 47.
- 5 Ibid, 44
- 6 Ibid, 44
- 7 Rizzo, Fundamentals, 30.
- 8 Ibid, 43
- 9 Hill, Cells, 48.
- 10 Ibid, 49
- 11 Edith Applegate, The Anatomy and Physiology Learning System (Philadelphia: Elsevier, Inc., 2006), 352.
- 12 Saltzman, Biomedical, Chapter 9, 6.
- 13 Kapit, Physiology, 58.
- 14 Ibid, 58
- 15 Ibid, 58
- 16 Ibid, 59
- 17 Ibid, 60
- 18 Ibid, 60
- 19 Applegate, Anatomy, 357.
- 20 Kapit, Physiology, 67.
- 21 Applegate, Anatomy, 358.
- 22 Rizzo, Fundamentals, 428.
- 23 Kapit, Physiology, 58.
- 24 Rizzo, Fundamentals, 428.
- 25 Saltzman, Biomedical, Chapter 9, 6.
- 26 Helmut G. Rennke, Bradley M. Denker and Burton David Rose, Renal Pathophysiology: The Essentials (Philadelphia: Lippincott Williams and Wilkins, 2007), 28.
- 27 'National Kidney Foundation', accessed August 10, 2011. http://www.kidney.org/
- 28 'Willem Kolff - Telegraph.' Telegraph.co.uk, accessed July 10, 2011. http://www.telegraph.co.uk/news/obituaries/4604625/Willem-Kolff.html
- 29 Saltzman, Biomedical, Chapter 16, 9.
- 30 Rennke, Renal Pathophysiology, 45.
- 31 Saltzman, Biomedical, Chapter 16, 9.
- 32 Ibid, Chap 16, 11
- 33 Eli A. Friedman and Mary C. Mallappallil, Present and Future Therapies for End-Stage Renal Disease (New Jersey: World Scientific, 2010), 149.
- 34 'Kidney Dialysis', University of Rochester, accessed August 17, 2011, lifesciences.envmed.rochester.edu/curriculum/SEPAClass/3.TEACHERKidneyDialysis7-23-09.pdf
- tabz alexander alo (kps, kabale, ug)
Subject taught: science, Grade: 1
good researcher
do osmosis take place in the kidney
THANK YOU — your feedback is very important to us! Give Feedback